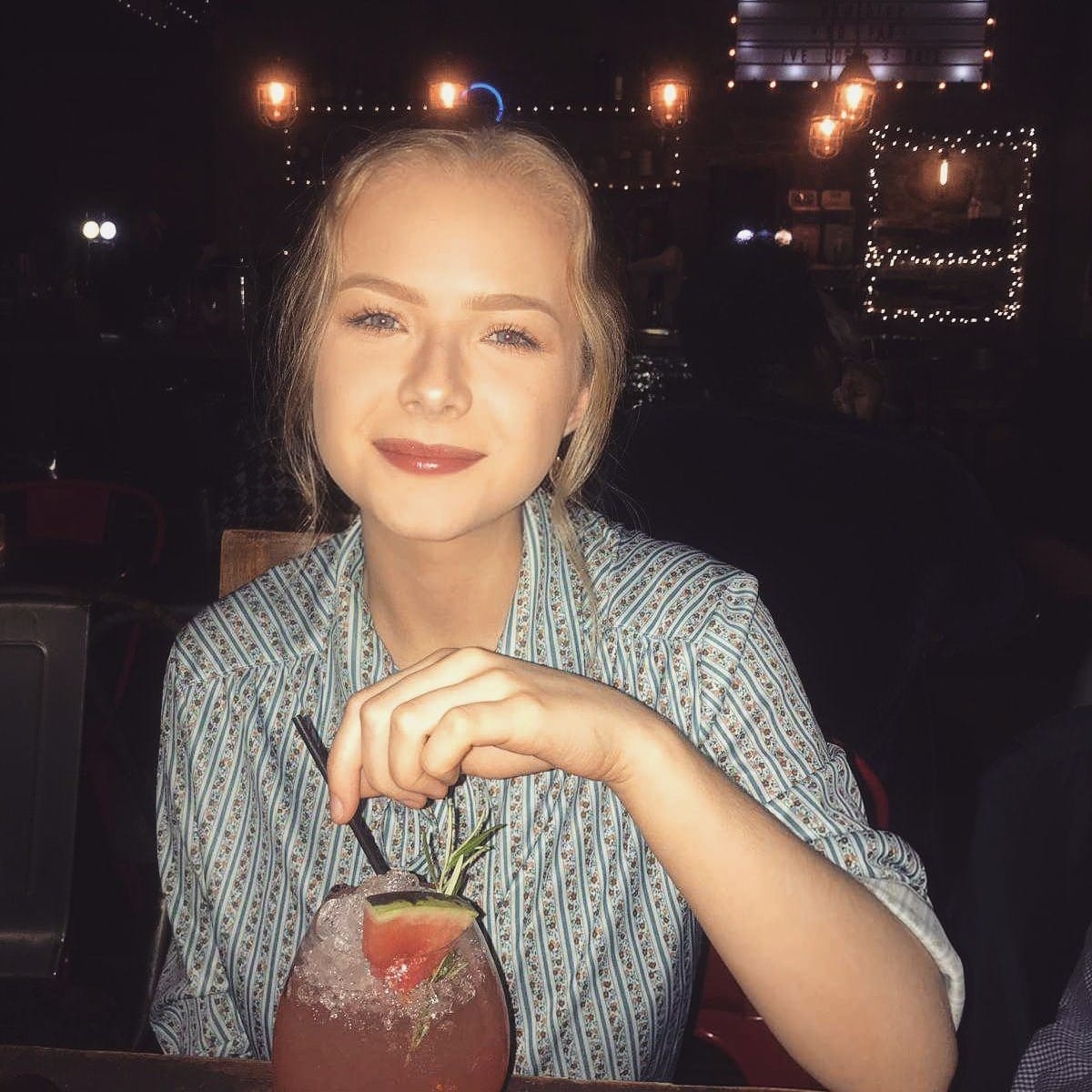
Two theoretical objects, crucial to the understanding of the birth and life of our universe, are perhaps unsurprisingly connected at fundamental levels: their apparent abundance in the early universe, pivotal role in galactic formation, and names which afford them qualities of mystery and intrigue before one even knows what they are. But what exactly are these objects, and how, exactly, are these connections made?
Supermassive black holes (SMBHs) are thought to exist primarily in the centre of galaxies, potentially as the seed of their formation. This theory is fuelled by their presence at high redshifts (i.e. very far away), where they are observed as ‘quasars’: bright objects existing in the very early universe that cannot be stars.
By definition, a black hole is an object with an escape velocity – that is, the speed with which you would have to travel to overcome its attractive gravitational force – greater than the speed of light. As nothing can travel faster than the speed of light, it follows that nothing can escape from inside a black hole. To be considered supermassive, such an object must weigh in at an equivalent mass of 1 million to 1.5 billion Suns.
Dark matter is less well-defined; believed to exist in copious amounts across the universe but remaining as of yet undetected. Its elusiveness is hypothesised to be the result of its component particle, a kind of weakly-interacting massive particle (WIMP), existing essentially unseen by anything on the standard model (our cumulative understanding of all particles and their interactions).
Nonetheless, its existence is well-supported by analysis of galaxy rotation curves, which would otherwise behave differently if the discs of galaxies were only comprised of ‘ordinary’ matter. This observation, and the requirement of an undetected matter component, was formally proposed by American physicist Vera Rubin in 1983, for which she never received a Nobel Prize.
Though we know comparatively little about it, dark matter is potentially a key component in the formation of SMBHs detected in the primordial (i.e. less than a billion years after the Big Bang) universe – those around which galaxies are thought to have evolved. If the theory is to be believed, so-called primordial black holes (PBHs) that formed from the collapse of dark matter congregations in a homogeneous universe are the seeds of these SMBHs that, by other established processes of black hole formation, could not have accreted their required mass of around a million solar masses in the given time frame.
Doubt is cast on this proposal, however, by observation the cosmic microwave background (CMB)’s blackbody spectrum, wherein no distortions expected to be caused by the presence of contemporary PBHs are detected; nor are the specific types of cosmic rays expected to be emitted by dark matter annihilation in the cores of the ultracompact minihalos (UCMHs) that would implicitly form wherever PBHs are found.
Whether dark matter plays a role in SMBH formation is therefore yet to be confirmed, and though connections in the early universe with sufficient evidence are yet to be made, their mutual contributions to the formation and structure of the galaxies we observe is not believed to be mere coincidence.
At sufficiently high redshifts (z ≳ 20), the universe is inhomogeneous: ordinary gases and dark matter travel at different relative – or streaming – velocities due to the former moving alongside massless photons and the latter being dictated predominantly by gravity. Around 700 million to a billion years after the Big Bang, at a redshift of z ≈ 7, the primordial universe can now be considered homogeneous, though matter-radiation equality is not yet established, and the streaming velocities of these two matter components continue to significantly influence the formation of early cosmological objects.
Currently unknown inflation models cause sufficiently large-amplitude (≳ 1mm) density perturbations to occur in areas of space, clumps of dark matter accreting until they exceed the Jeans mass of around 10 million solar masses and incur cumulative gravitational collapse. In areas of large streaming velocities, the resulting star formation expected to occur in these overdensities could potentially be supressed – allowing for direct collapse into the PBHs that could accrete mass and/or combine to form the SMBHs observed at high redshifts. Interestingly, this same mechanism also prevents or at least makes it more difficult for relatively light and distant dark matter halos over relatively small (but still intergalactic) scales to accrete gas and undergo collapse.
Formed alongside PBHs are UCMHs of dark matter, which grow slowly when radiation dominates and relatively rapidly when matter dominates. All galaxies form within these dark matter halos, and for a galaxy to be formally classified as such it must not have collapsed directly into a black hole as in the previous scenario, but instead accommodate star formation; the first of these are powered by dark matter particle annihilation in the core and thus are known as dark stars.
The dark matter annihilation process is analogous in this context to nuclear fusion in later stars, in that it provides a means for hydrostatic equilibrium and the prevention of gravitational collapse, though, as in later stars, this cannot be maintained indefinitely – most dark stars are believed to achieve masses that make them credible candidates to collapse into intermediate-mass black holes or potentially SMBHs.
The candidacy of PBHs as high-redshift SMBH seeds, however, is highly contested by non-observations of the CMB’s blackbody spectrum. The lack of distortion, which would be expected as a result of the presence of PBHs, can exclude such objects of mass greater than around 100 000 solar masses, hence casting a great deal of doubt on their role in the formation of the SMBHs believed to be observed.
Smaller PBHs can potentially be excluded by another means: when observing UCMHs, it is expected that cosmic rays (e.g. high-energy gamma photons or neutrinos) will be detected due to WIMP annihilation in their dense dark matter cores. The signals that are received, however, have inconsistent properties with those expected if it is assumed that the associated PBHs will evolve into SMBHs – namely the interaction strengths of these particles with those on the standard model.
Whether dark matter plays a role in SMBH formation is therefore yet to be confirmed, and though connections in the early universe with sufficient evidence are yet to be made, their mutual contributions to the formation and structure of the galaxies we observe is not believed to be mere coincidence. In the future, physicists hope to confirm the existence of SMBHs – particularly that thought to lie in the centre of our own galaxy – as well as make confirmed observations of dark matter particles that may help unlock the secrets of their role both in galactic structure and in the wider universe.
Abbie Rhodes